
Creating the hydrogen highways of the future requires economical, efficient, and safe hydrogen
storage. High-pressure gaseous hydrogen storage, at pressures up to 100 MPa, offers the simplest and most popular solution in terms of infrastructure and transportation requirements. High-pressure hydrogen creates new, immense technical challenges for pressure vessel designers and manufacturers. This is a young market with plenty of room for technology innovation and cost reduction.
In expensive cities like Tokyo and Seoul, hydrogen fueling stations must be as small and efficient as possible. Total station costs include the high cost of land combined with the cost of the capital equipment. The method for refilling the hydrogen storage tanks must be quick and unobtrusive to prevent disruptions to local traffic flow. The size and pressure of hydrogen storage must be carefully limited to reduce casualties and damage in case of a potential safety incident.
From the pressure vessel standpoint, “low cost” and “high pressure hydrogen” present a difficult combination. Each type of materials used in hydrogen pressure vessels have their own challenges.
TYPE 1 and TYPE 2 STEEL VESSELS: It’s all about fatigue cracking.
Type 1 steel vessels are standard homogenous steel without any additional reinforcement. Type 2 steel vessels have steel liners that are reinforced in the hoop direction by carbon fibers, glass fibers, or steel wire.
The high strength steels used in high-pressure tubes are susceptible to hydrogen embrittlement during service, causing fatigue cracks to grow quickly during service. Special manufacturing techniques, testing methods, and fracture mechanics analyses are required to design and build these pressure vessels properly.
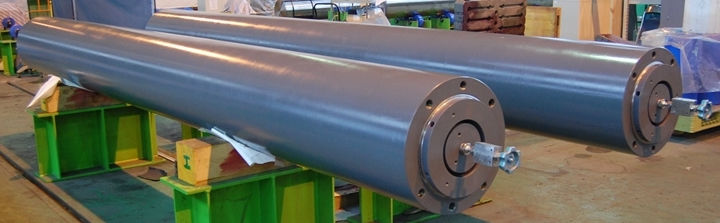
Testing data, analysis methods, and industry standards were developed by industry pioneers Sandia Laboratories, Powertech Laboratories, US Department of Energy, CSA Canadian Standards Association, FIBA Technologies Inc., Japan’s JSW, Japan’s HYDROGENIUS group, and others. From this work, new industry standards were created, such as ASME Section VIII, Division 3, Article KD-10.
ASME Section VIII, Div 3, KD-10 requires special techniques to be employed by pressure vessel manufacturers. Features of particular importance include the proper selection of steel alloy and heat treatment method. Internal surface finish must be nearly flawless, in the sidewall section and the dome regions. Finite element analysis and special fracture mechanics analysis are required to determine the estimated time for a flaw to reach critical crack depth for each range of pressures that are anticipated during use.
Manufacturers of Type 1 and Type 2 hydrogen pressure vessels include FIBA Technologies, Wire Tough Cylinders, CP Industries, JSW of Japan, and others.
TYPE 3 CYLINDERS: Fatigue life of the aluminum liners is key.
Japan’s Samtech and America’s Worthington Industries SCI group were pioneers in Type 3 composite hydrogen cylinders, aluminum liners with full wrap carbon/epoxy composite structural wrap. Industry standards for design and manufacturing include ISO11119-2.
Aluminum liners are not affected by hydrogen embrittlement. However, the technology is still challenging. The very high service pressures, 50 MPa, 70 MPa, and 900 MPa impose massive challenges for attaining the proper pressure cycle life and burst strength. The aluminum liners must have very fine surface finish, very high strength and toughness. Fatigue life of the aluminum liners often depends on the quality of the composite reinforcement wrap. The composite laminate wrapping sequence, fiber tensions during winding, cure cycle, and autofrettage pressure must be carefully calculated and controlled. Due to manufacturing techniques and machines, Type 3 cylinders are limited in size to approximately 670 mm diameter by 3 meters long.
TYPE 4 COMPOSITE PRESSURE VESSELS: Permeation, neck connections, and cost are key components to success.
Type 4 composite pressure vessels have polymer liners that are reinforced with full wrap of carbon
and/or glass fiber composite. Type 4 composite pressure vessels offer flexibility in size, with diameters up to 1.4 meters and lengths up to 10 meters or longer. Pioneers in development of Type 4 hydrogen storage vessels include Hexagon Composites and Quantum Fuel Systems. ASME Section X, Division 3 is one of the regulatory standards developed to address Type 4 composite pressure vessels for stationary hydrogen storage.
Permeation of hydrogen through the polymer liner is a key concern because hydrogen atoms are very small. HDPE is a common liner material for storing hydrogen at pressures up to 50 MPa.
Pressures above 50 MPa typically require other materials with much lower permeation, such as polyamides PA-6 and PA-12. Creating a leak-proof connection between the polymer liner and metal neck, known as polar bosses, is a critical challenge as well. Most manufacturers have developed their own specialized and patented polar boss connections.
Creating low cost, efficient composite wrap with consistent strength is the biggest challenge. Large diameter, thick composites often end up with wrinkled fibers and high residual stresses. Attaining the desired strength, pressure cycle life, and tight statistical consistency are major challenges in cost reduction efforts.
Want to learn more? Contact the companies listed in this article for more detailed information on their hydrogen storage vessels. And, of course, contact Hansho Composites for expert advice, design and testing, or other technology services.